VIOLIN ADVISOR
Stewart Pollens, Director
"Violin Advisor assists in the acquisition of investment-grade violins.
Enjoy solid growth plus the privileges of arts patronage." - Financial Times
The following talk was given by Stewart Pollens at a joint meeting of the Galpin Society, the Institute of Acoustics, and the Royal Musical Association on September 29, 2015.
When Science Goes Bad
​
In recent years, those interested in the violin have reached out to physicists, chemists, engineers, and dendrochronologists to answer questions about the violin’s acoustics, the composition of its varnish, and to assist with dating, authentication, and establishing condition. In addition to responding to such inquiries, scientists themselves have been sufficiently intrigued about the violin to initiate their own independent studies. However, in evaluating published findings, we must be aware that there is “good science” and “bad science.” In some instances scientific tests may be flawed or inappropriate, and in other cases unsupportable or exaggerated conclusions may be drawn. Sadly, the peer-review process does not guarantee the validity of published research; in fact, peer review is sometimes sidestepped due to the intense interest such studies provoke. This was the case when I was asked to review an article submitted to Chemical & Engineering News in 1988 by Professor Joseph Nagyvary of Texas A&M University. Despite the major flaws that I pointed out in my review, the editor (David M. Kiefer) sent me the following reply: “Thank you for your very thorough, thoughtful, and informative review of the article by Joseph Nagyvary dealing with his research on violins. Despite your strong misgivings, we decided to publish the article. However we did modify it to some degree on the basis of what you had to say. . . . We also added a short editorial comment—something in the nature of a disclaimer—that is drawn to some degree from your critique. . . . Our special reports typically have a stronger scientific foundation; however, I think that our readers will find Nagyvary’s exploration . . . a change of pace for us and both interesting and thought provoking. Many thanks, again, for your fine efforts on our behalf and for the time and careful thought you put into your review.” I should add that I was recently asked to review another article by Nagyvari that was submitted to the Journal of the Violin Society of America. The editor and I went through the same rigmarole, and at the end of the day, my suggestions were used to superficially improve another of Nagyvary’s questionable articles.
Analysis of materials
In 2009, working with the Department of Scientific Research at the Metropolitan Museum and McCrone Associates in Chicago, I employed gas chromatography to analyze five samples of Stradivari’s varnish. The analysis indicated that his varnish consists primarily of linseed oil and pine resin (see The Strad; May, 2009; and Pollens, Stradivari, 2010). Raman spectroscopy identified a red inorganic pigment dispersed in Stradivari’s varnish as red ochre. Inorganic materials can also be identified with a scanning electron microscope equipped for energy-dispersive x-ray analysis (termed “EDX”). In 2000 I used such a scanning electron microscope to analyze the silver used in the fittings of a Francois-Xavier Tourte bow. I discovered that the alloy of silver he used to make the ferrule and back plate of a frog and the rings of a button consists of 88.7% silver and 11.3% copper, precisely the same composition as a contemporary French écu coin minted in 1781. This suggests that the silver he used was hammered or rolled into sheets from coins then in circulation. These are examples in which the appropriate analytical techniques were employed in identifying organic and inorganic materials.
However, in 1988, using an electron microscope equipped with EDX, Professor Nagyvary claimed to have discovered a layer of crushed rubies, garnets, and other gemstones beneath the varnish of one of Stradivari’s violins, and published this in Naturwissenschaften and Chemical & Engineering News. Unfortunately, these findings must be challenged because EDX only detects discrete elements, not molecules, and it does not reveal crystalline structure, which is critical in identifying gemstones. Other researchers, C. Y. Barlow and J. Woodhouse of Cambridge University also claimed to have discovered particles under Stradivari’s varnish using similar equipment, though they suggested that these particles were volcanic ash due to the presence of silicon, potassium, and titanium. They published their findings in the Journal of the Catgut Acoustical Society in 1989. I should mention that EDX equipment used in the 1980s could not detect elements lighter than sodium; lighter elements such as carbon and hydrogen, which make up over 98% of wood and varnish, completely eluded that earlier equipment; thus the elements they identified might have been trace elements in the wood or varnish. Today’s equipment can detect elements as light as carbon. Other techniques should have been employed in identifying the particles these researchers claim to have discovered—for example, a polarized-light microscope could have been used to characterize their optical properties and thereby identify them conclusively. Note in the epi-fluorescence photomicrograph shown below that no particulate layer can be seen beneath the two layers that comprise this cross section of Stradivari’s varnish; the filters that I employed would have caused such crystalline matter to fluoresce brightly. I should add that I have never observed a layer of crystalline particles in any samples of Stradivari’s varnish that I have studied.
​
Acoustics
The science of musical acoustics has advanced significantly in the twentieth century with the development of the audio-spectrum analyzer, the high-speed computer, hologram interferometry, and the application of mathematical techniques such as Fourier transform, constant Q transform, and finite-element analysis. The acoustical properties of violins are highly dependent upon the way they are setup in the laboratory and activated when measurements are made—some physicists use mechanical bowing devices, others drive the bridge by use of a magnet and voice coil, while others stimulate vibrations in the instrument with a speaker. These results vary to such a degree that physicists cannot even agree whether the violin behaves like a dipole or monopole radiator.
​
MIT study
A paper entitled “The Evolution of Air Resonance Power Efficiency in the Violin and Its Ancestors” by a group of researchers at the Massachusetts Institute of Technology was published in the Proceedings of the Royal Society on February 11, 2015. This paper sparked a debate in the media and stringed instrument world, primarily with regard to their contention that “by [biological] evolution-rate analysis . . . changes [in violin f-hole length] are found to be consistent with [biological] mutations arising within the range of accidental replication fluctuations from craftsmanship limitations and subsequent selection favoring instruments with higher air-resonance power.” In plain English, these researchers claim that inadvertent slips of the knife, rather than intelligent design, were responsible for a gradual lengthening of the violin’s f-holes and a concomitant increase in acoustical power.
While, as the authors of the MIT study assert, it may be true that minor dimensional differences in the shape of f-holes are due to the vagaries of handcrafting, the elongated shape of Joseph Guarneri del Gesu’s late f-holes, for example, is certainly an intentional stylistic departure from the norm, rather than an “accidental fluctuation in craftsmanship,” as the MIT study claims.
​
The other conclusion of these researchers is that f-hole length is the primary factor governing the “acoustic power” of violins, and that f-holes steadily increased in length from the Amatis through the death of del Gesu in 1745, which the researchers consider the culmination of the violin’s evolution. These researchers dismiss plate thickness and arching as of secondary importance. In fact, flat arching is generally associated with a more powerful tone, and del Gesu violins with pancake-flat tops and f-holes of conventional length (such as the 1733 “Kreisler”) are among the loudest of his violins.
The effect of f-hole dimensions on Helmholtz resonance is, in fact, well known—it is not a discovery of the MIT group. Basically, a Helmholtz resonator is a chamber with an opening (in this case, the violin’s body is the chamber and the f-holes are the opening). The volume of the chamber and the dimensions of the opening affect the resonant frequency of the system. Within certain limits, larger openings lower the resonant frequencies of chambers. The MIT researchers studied the f-hole’s effect on acoustical power at the violin’s Helmholtz resonance, which is around 290 Hz, or the pitch of an open D string. In an acoustical experiment that I did back in the late 1970s, I plugged up the f-holes of a Stradivari copy with cotton wool, and noted that the violin’s resonance at that frequency was greatly diminished, whereas the resonances at higher frequencies were relatively unaffected. In attempting to verify their contention that a 30% increase in f-hole length corresponds to a roughly 60% increase in acoustic power, I measured the sound output of a Guarneri copy and then shortened the f-holes by 30% by covering part of the holes with drafting tape. I used a force gage to pluck the violin’s open E string tuned to 659 HZ at constant force with and without the altered f-holes, and I could not detect a difference in volume using a decibel meter. While F-hole length may be a factor at 290 Hz, the musical sounds produced by a violin include tones and harmonics ranging into the thousands of Hz, and it is the sound production in that extended region that establishes the tonal characteristics and power of an instrument.
As outlined by the MIT researchers, the history of f-hole length is simplistic and historically inaccurate. Their graph of the growth of f-hole length over time represents only the work of the Amati, Stradivari, and Guarneri families, and terminates the year that Guarneri del Gesu died, 1745. Thus this graph is skewed by about a dozen frankly eccentric instruments made by del Gesu in the last two or three years of his life. Had they included makers besides those of the Amati, Stradivari, and Guarneri families, and beyond 1745, f-hole length would revert to the mean, and the lengths of late del Gesu f-holes would just be one of many blips on the curve rather than the termination point.
​
The Fritz study
Claudia Fritz’s so-called “double-blind” psychoacoustic study was conducted under the aegis of the National Center for Scientific Research in France (Centre national de la recherché scientific in France). It was published in the Proceedings of the National Academy of Science (US) in 2014 and widely discussed in the press. It ostensibly demonstrated that professional violinists cannot tell the difference between multi-million dollar Stradivari and Guarneri violins and cheap modern ones.
In the Fritz test, each violinist was given a total of 50 minutes to evaluate and rank 12 instruments, and during that time the violinists had the option of getting feedback from a designated listener and to have someone play the violins for them—in other words, the violinists may have spent considerably less than 4 minutes actually playing each violin. They then had another 12 minutes to evaluate a selection of their favorites, and finally 30 seconds to test each of their four finalists. Firstly, the “old violins” that took part in this study were lent under the condition of confidentiality, so we do not know for a fact whether these alleged Strads and Guarneris were authentic, or anything about their condition. Furthermore, it is extremely unlikely that those violins were setup to suit the personal tastes of the individual violinists. Setup is a time consuming process that may involve installing different strings, moving the soundpost, fitting a new bridge, and even resetting the neck. I can report that shortly after Itzhak Perlman acquired his 1714 Stradivari violin in 1986, he admitted to me that he did not particularly care for it and that he had acquired it primarily because it had been owned by his boyhood idol, Yehudi Menuhin, and secondarily as an investment. However, after some adjustments were made to the violin and a period of acclimation transpired, he came to favor it and has been using it as his primary instrument ever since. Thus, I submit that the brief time allotted to the players in the Fritz study was insufficient for them to evaluate the instruments set before them.
​
Determination of age
There are several scientific methods of dating wood, the most often cited being radiocarbon dating and dendrochronology. Radiocarbon dating does not yield a precise date, but a range of ± 80 years. While this may be adequate for distinguishing a Gasparo da Salo violin from a nineteenth-century copy, it is not precise enough for the type of dating we need to authenticate most violins. Dendrochronology relies upon the correlation of yearly weather conditions with the growth of certain trees—in other words, if there was plentiful rainfall and good sunlight one year, a tree’s year-ring would be wide, and with poor weather conditions the next year, its corresponding year-ring would be narrower. These ups and downs can be compared against master chronologies from different regions, and when there is a 65% or greater match, the precise date of each year ring is ostensibly determined.
What does dendrochronology tell us? It is a generally held misconception that the last dated tree ring corresponds to the year the instrument was made. This is not true: the date of the last year-ring in a sequence merely indicates that the instrument could not have been made before that date because the tree was still alive in the forest, though it could have been made any time later. Unlike radiocarbon dating, the results obtained from dendrochronology are potentially accurate to the year, but there is a statistical component to this dating procedure, and herein lies the dirty little secret about dendrochronology, which is, that most workups do not arrive at a single match but with several matches having similar levels of statistical significance, and these alternate dates typically do not fall close to one another—they may be many decades or even hundreds of years apart.
Furthermore, various mathematical manipulations of the raw data (such as indexing, removal of autocorrelation, use of running means, segmentation, etc.) often yield wildly different results. Thus, one dendrochronologist’s conclusion may differ from that of another, as was the case with the “Messiah” violin, which is widely believed to be the finest and most perfectly preserved specimen of Stradivari’s work.
However, in 1998, I demonstrated that the long-standing provenance of the “Messiah,” which ostensibly linked it to Stradivari’s shop, was fictional, and I proved that a critical inscription in the violin’s pegbox, thought to be in Stradivari’s hand, is a twentieth-century forgery. Because of these discoveries, I sent year-ring data from the “Messiah” to Dr. Peter Klein, a noted dendrochronologist at the University of Hamburg, who determined that the last year-ring of that violin was 1738, which not only post-dated the putative date of the violin but also the year of Stradivari’s death. Klein’s findings were confirmed by another noted dendrochronologist, Dr. Peter Ian Kuniholm of Cornell University. I should add that both of these dendrochronologists were not told what violin they were dating.
The fact that the “Messiah” was proven to be a fake was obviously unacceptable to the violin trade, and though my findings regarding provenance and the falsified inscription were swept under the carpet, the date discovered by Klein and confirmed by Kuniholm had to be refuted. A British violin maker and amateur dendrochronologist named John Topham stepped forward and claimed to have discovered an earlier date, 1682, which was consistent with the putative date of the “Messiah,” 1716. The non-standard technique that he employed made use of a so-called “floating chronology” derived from two other instruments attributed to Stradivari. This floating chronology was ostensibly fixed in time by cross-dating it with a master chronology, but that floating chronology only extended to 1685, which prevented Topham from discovering a later date having a better statistical match.
The Violin Society of America decided to resolve the issue of the two conflicting dates by engaging Dr. Henri Grissino-Mayer, a dendrochronologist at the University of Tennessee. In his preliminary workup using a public-domain master chronology from the alpine region, he admitted that he had confirmed Klein’s findings, but for some reason he decided to reject that date. Though one would assume that he would work independently of Topham, the two teamed up at the Ashmolean Museum, and Grissino-Mayer adopted Topham’s “floating chronology,” thereby arriving at precisely the same date as Topham. They published together in the journal Dendrochronologia in 2009.
In an article on the dendro-dating of the “Messiah” published in the Journal of the Galpin Society in 2002, Topham is quoted as having stated that the wood used to make the “Messiah” probably came from the Oetztal Alps in Austria. This statement intrigued me, so I compared the year-ring measurements of the “Messiah” with a master chronology from that region, the Siebenlist-Oetztal chronology, and found that the date of 1682 achieves a match of 47% for the violin’s treble side and 52% for the bass side—neither of which remotely qualifies as a match, whereas there is a very compelling 65% match for the date 1774.
In conclusion, I would strongly suggest that “scientific studies” of musical instruments, and especially those involving the violin, be given great scrutiny, even when they have been conducted at great institutions of learning, underwritten by august societies, and published in respected journals and museum catalogues.
Ultra-violet fluorescence photomicrograph of a cross section of a chip of wood from a Stradivari cello dated 1736 showing two distinct layers of varnish applied directly to the wood. No particulate ground can be seen in this or other samples studied by Stewart Pollens.
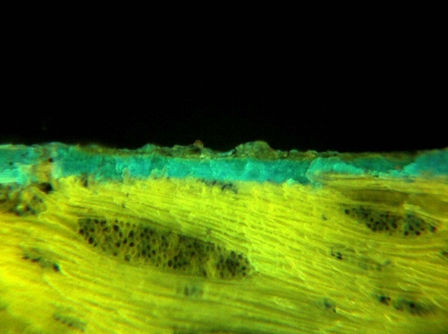